What is permafrost and how does it impact climate change?
The Arctic is particularly affected by climate change. Rapid warming results in the thawing of permanently frozen ground, known as permafrost. The remains of dead plants and animals have been frozen in the permafrost for hundreds of thousands of years. When permafrost thaws, bacteria begin to decompose these remains. This releases the greenhouse gases carbon dioxide and methane, which further accelerate global warming. Permafrost soils contain around 1500 gigatons of organic carbon. This is roughly twice the amount that is currently in the atmosphere in the form of carbon dioxide. Thawing permafrost soils could release tens to hundreds of billions of tons of organic carbon until the year 2100. This would drastically accelerate climate change.
How much greenhouse gases can humans still emit or how much carbon must be removed from the atmosphere to limit global warming to 1.5°C above pre-industrial levels? There is widespread agreement that the 1.5°C limit can just about prevent a dangerous disruption to the climate system. If the 1.5-degree limit is exceeded, extreme weather and other climate impacts would be almost impossible to cope with and would cause the economic costs to rise unacceptably high. Greenhouse gas balances from models do not yet account for how permafrost landscapes will change with the future climate. Permafrost ecosystems are characterized by complex interactions between climate, water, organisms, and soil, many of which are still unexplored. Therefore, future greenhouse gas balances are still highly uncertain.
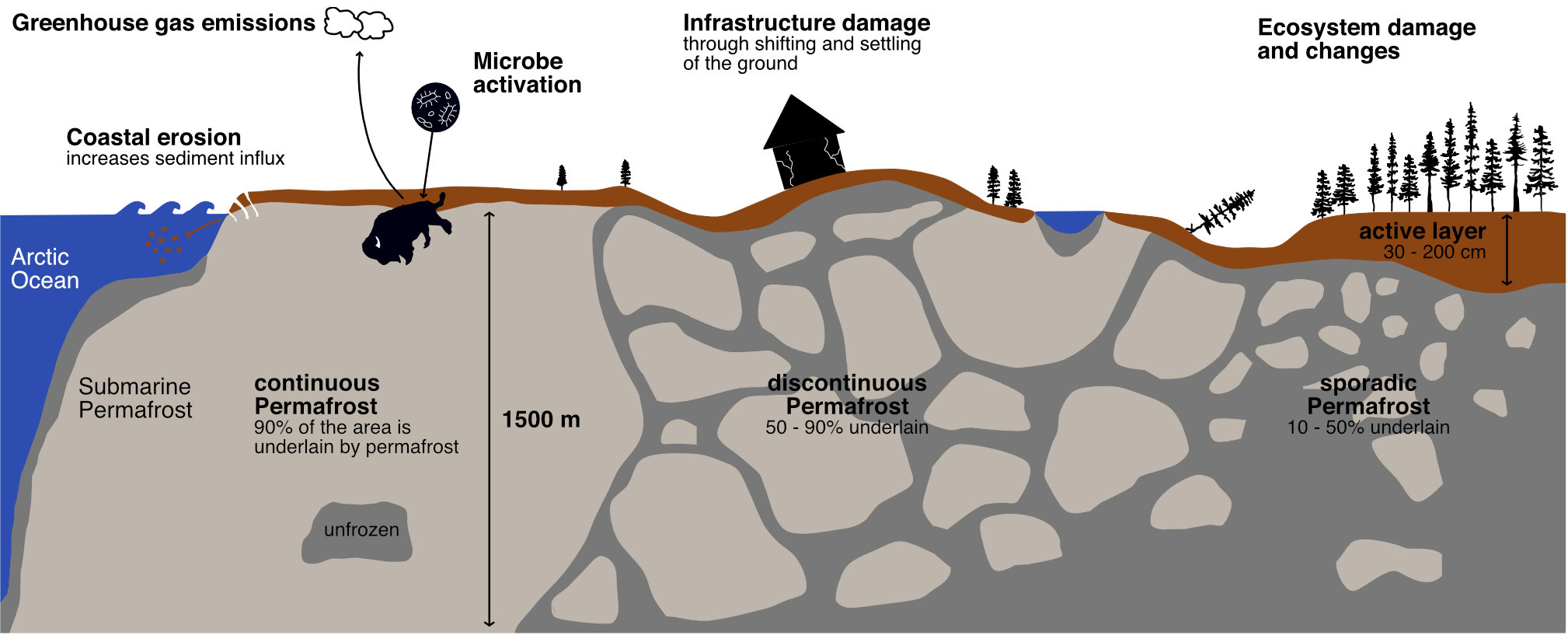
What happens when permafrost thaws?
Permafrost soils cover a fifth of the Earth’s land surface and are mainly found in the northern hemisphere (mainly in Siberia, Canada, Alaska, China and Greenland, but also at high altitudes in the Alps). Most of today’s permafrost was formed during or since the last ice age 100,000 to 10,000 years ago.
Soils in permafrost regions typically consist of two layers: a thin layer up to two meters deep, which thaws in summer and freezes again in winter, the so-called active layer, and the permanently frozen permafrost layer underneath.
The permafrost layer can be several meters to several hundred meters thick. This depends on the air and soil temperature, the properties of the soil (especially the water content), and the density of the vegetation. In parts of northern Siberia, for example, there is extreme cold in winter and very little snowfall. Without the protective snow cover, the cold can penetrate deep into the ground, so that the permafrost can reach around 1500m into the earth’s interior.
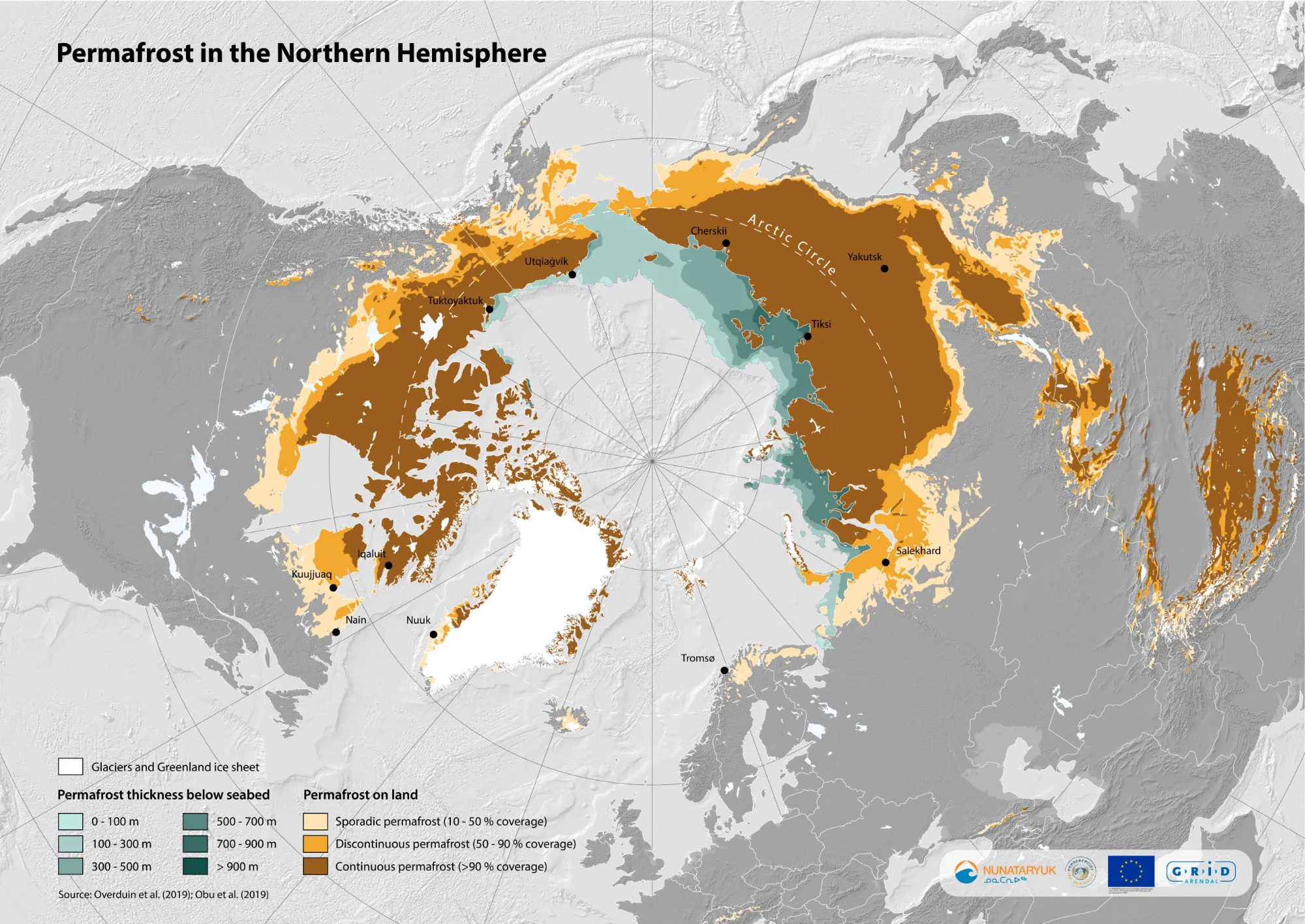
Permafrost consists of rock, sediment, and soil with varying amounts of ice, which acts like cement and holds the loose sediment together. When this ground ice melts, the ground becomes weak and unstable. This can result in a wide range of damages:
Infrastructure damages
In cities built on permafrost soils, the infrastructure was constructed assuming that the solid foundation of the permafrost would not change. Decreasing soil stability and shifting and settling of the ground cause direct damage to buildings, roads, pipelines, industrial facilities, and other infrastructure. This affects around 4.9 million people. Over the next few decades, enormous costs will arise to combat and mitigate the damage. Disruptions to everyday services, relocation, and repair costs will require hundreds of millions of dollars.
Ecosystem damages
Permafrost is impermeable to water. An increase in permafrost temperature therefore significantly impacts water conditions in the soil and on the surface. The loss of permafrost under water bodies can cause the water to seep through the ground. This results in the loss of countless lakes and wetlands and can also jeopardize the drinking water supply for settlements.
Shifting and settling of the ground due to thawing permafrost creates an uneven hilly landscape known as thermokarst. Water collects in the depressions and forms lakes, while the hills dry out. As a result, the changes in the permafrost also cause changes in the vegetation. If the soil loses stability, tree roots are damaged and trees are uprooted. In areas that have dried out, the risk of forest fires and insect infestation increases, while trees in newly formed depressions can die due to waterlogging.
During the thawing phase in summer, wetlands and lakes can accelerate the thawing processes of the permafrost beneath them, as they conduct heat better. In winter, on the other hand, they act as an insulating layer so that the ground below freezes more slowly. Permafrost thawing and lake development are therefore mutually reinforcing processes.
Another problem is that thawing permafrost releases sediments and pollutants such as mercury, which get transported into the Arctic Ocean via rivers. These are harmful to the environment and can enter the marine food chain.
The rapid landscape changes also pose major challenges for animals and can lead to species loss.
Coastal erosion
Unstable, weakened coastlines, which are further weakened by receding sea ice, warmer waters, and longer storm seasons, are more susceptible to erosion. This threatens coastal settlements. Coastal erosion also releases sediment, additional nutrients, and carbon into the ocean, contributing to ecosystem change and ocean acidification.
Landslides
At high altitudes and in steep mountainous terrain, the thawing of permafrost increases the risk of rockfalls and landslides, which can cause additional hazards.
What is research working on?
Thawing permafrost threatens the global climate and poses major challenges for the local population. However, intelligent land use concepts have the potential to reduce permafrost thawing, greenhouse gas emissions, and species loss and thus make it easier to achieve the United Nations’ sustainability goals. To better understand how climate change affects permafrost ecosystems and, conversely, how changes in permafrost ecosystems affect climate change, German polar research is focusing on the following questions:
What are the impacts of climate change on permafrost, the dynamics of permafrost landscapes, and future greenhouse gas emissions?
Arctic permafrost landscapes with high levels of ground ice are particularly at risk from global warming. As the ground ice melts, it leads to rapid changes in the landscape, such as thermokarst and erosion. Permafrost sediment archives provide evidence of this also for past warming phases. The rapid warming of permafrost as well as additional disturbances caused by thermokarst lakes, fire, or erosion can lead to serious changes in permafrost ecosystems within years to decades. Observations on the ground and satellite-based observations as well as numerical modeling provide evidence of the landscape changes over large regions. This is accompanied by the largely irreversible change in water conditions and biogeochemical processes. Reliable insights about changes in permafrost landscapes and future greenhouse gas emissions require long-term observations of permafrost temperatures, soil properties, and greenhouse gas emissions.
How do vegetation, biodiversity, permafrost and the climate interact, and what changes occur in ecosystem functions?
The complex interactions between thawing permafrost, microorganisms, nutrients, and plants leading to the release of greenhouse gases are not yet fully understood. Therefore, future greenhouse gas balances are still highly uncertain.
While methane emissions will rise sharply in areas that are becoming wetter, increased carbon dioxide emissions are expected in areas where soils are becoming warmer and drier. There are still major uncertainties in estimating methane emissions from the numerous lakes and wetlands of the permafrost regions and from adjacent shelf seas. For this, there is not enough data available yet. Initial studies indicate the significance of greenhouse gas emissions in the cold seasons from October to May. These may have already led to permafrost areas becoming a source of carbon.
It is increasingly recognized that biological feedback mechanisms can lead to the stabilization of permafrost ecosystems. Siberian larch forest, for example, has a flat, dense network of roots which is insulating the ground ice. As a result, the permafrost thaws more slowly there than in non-forested areas. It also delays the immigration of competitive evergreen conifers, which store more heat radiation due to their dark surface.
What influence do rising sea temperatures have on the stability of submarine permafrost and the associated carbon storage?
In large areas of the shallow Siberian shelf seas, there is submarine permafrost beneath the sea floor. It formed during the last ice ages when the sea level was around 120 meters lower than it is today. The thickness of the submarine permafrost ranges from a few tens to around 800 meters deep. It is still unclear to what extent climate change has an impact on the stability of the submarine permafrost.
Long-term oceanographic measurements in the central Laptev Sea have shown a significant increase in surface water temperature by an average of 4 °C in summer since 2005. The average water temperature at the sea floor is around -1.3 °C. However, warmer periods of up to 0 °C are reoccurring. These periods have become longer and warmer in recent years. More data is needed to clarify how the increasing surface water temperatures affect the temperatures at the bottom.
It is also unclear what role microorganisms play in the formation, stabilization, and degradation of gas hydrates. Gas hydrates are crystalline, ice-like solids consisting of a gas such as methane or carbon dioxide and water. It is assumed that unfrozen zones in the submarine permafrost are transport routes for gases released from gas hydrates. The release of methane deposits that have been trapped in the submarine permafrost for thousands of years would have a significant impact on the global climate.
How do changes in permafrost ecosystems and Earth system feedbacks affect the achievement of UN sustainability goals?
Changes in permafrost ecosystems can exacerbate human-induced climate change. The extent to which additional greenhouse gas emissions from permafrost soils will contribute to climate change depends primarily on what measures are taken now to reduce global warming.
Models can be used to estimate the additional emissions for different scenarios. These emissions will be partially offset by increased plant growth in a warmer Arctic. However, the immigration of shrubs and forest into the tundra will also lead to additional warming, as they darken the land surface and can therefore absorb more heat radiation. A better understanding of the processes in permafrost landscapes is necessary to achieve the United Nations Sustainable Development Goals 13 – “Climate Action” and 15 – “Life on Land”. This requires more observations and a stronger integration of models and observations.
Permafrost-climate feedback is a threat to the stabilization of the climate because thawing processes, once set in motion, are not reversible and can continue to have an effect for hundreds of years after the end of human greenhouse gas emissions. The future costs of reducing climate change will be particularly high in this case.
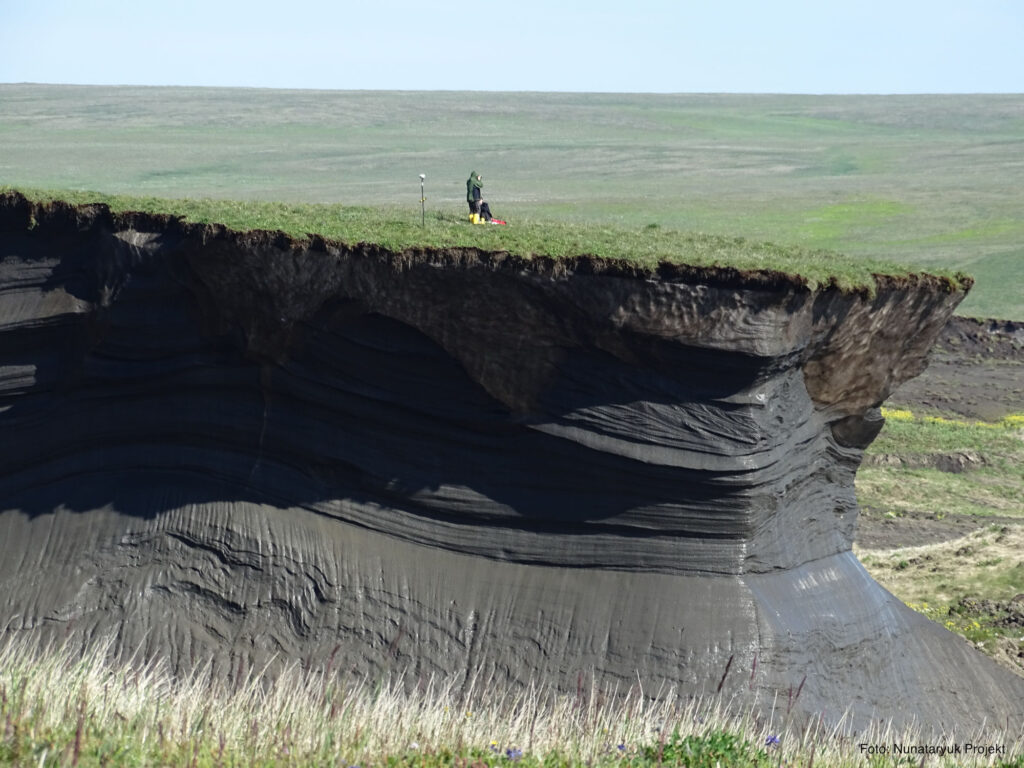